Where Does Gold Come From? NASA Data HasClues
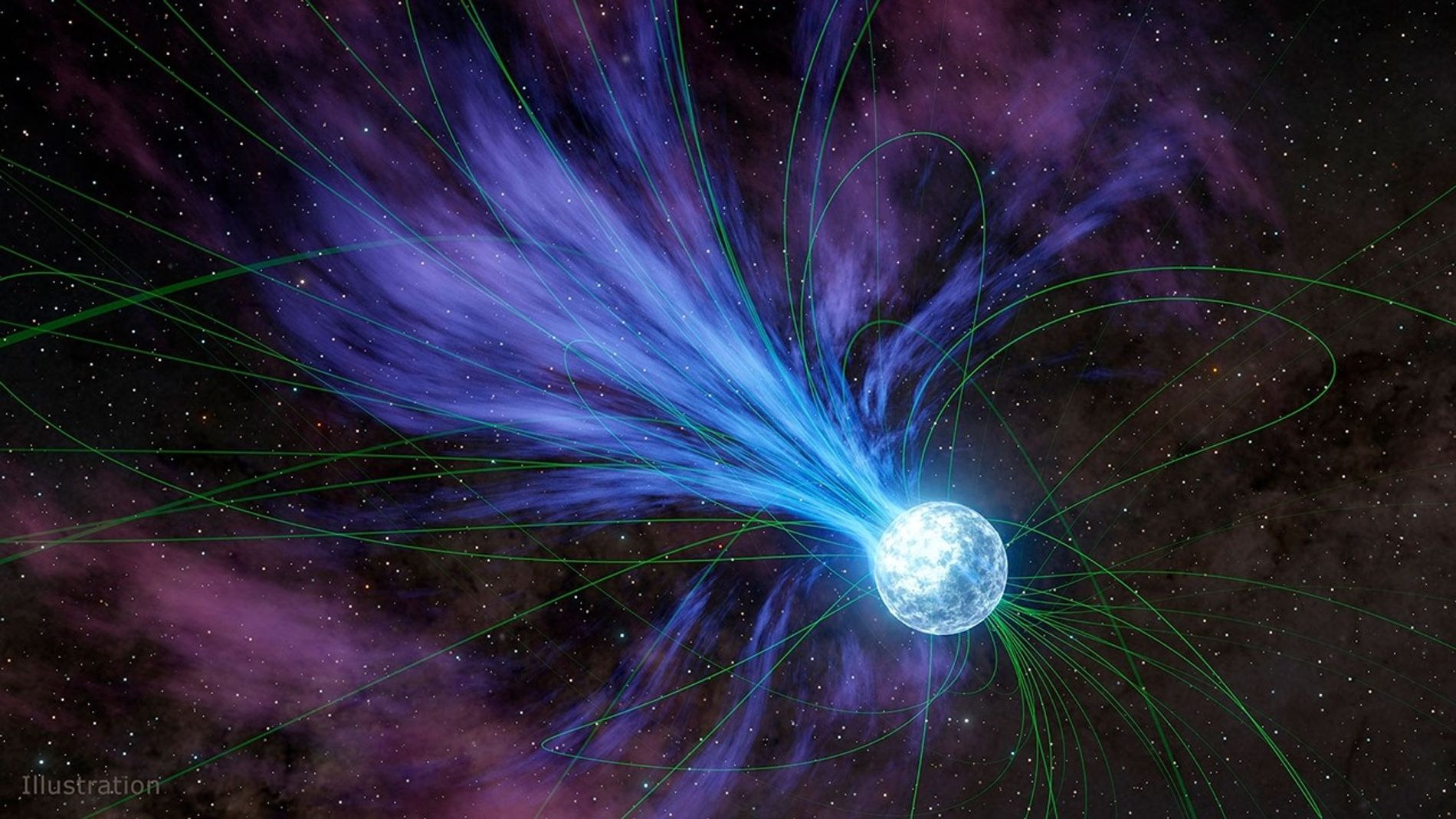
This artist’s concept depicts a magnetar – a type of neutron star with a strong magnetic
field – losing material into space. Shown as thin green lines, the magnetic field lines
influence the movement of charged material around the magnetar.
NASA/JPL-Caltech
Since the big bang, the early universe had hydrogen, helium, and a scant amount
of lithium. Later, some heavier elements, including iron, were forged in stars.
But one of the biggest mysteries in astrophysics is: How did the first elements
heavier than iron, such as gold, get created and distributed throughout the
universe?
“It’s a pretty fundamental question in terms of the origin of complex matter in the
universe,” said Anirudh Patel, a doctoral student at Columbia University in New
York. “It’s a fun puzzle that hasn’t actually been solved.”
Patel led a study using 20-year-old archival data from NASA and ESA telescopes
that finds evidence for a surprising source of a large amount of these heavy
elements: flares from highly magnetized neutron stars, called magnetars. The
study is published in The Astrophysical Journal Letters.
Study authors estimate that magnetar giant flares could contribute up to 10% of
the total abundance of elements heavier than iron in the galaxy. Since magnetars
existed relatively early in the history of the universe, the first gold could have
been made this way.
“It’s answering one of the questions of the century and solving a mystery using
archival data that had been nearly forgotten,” said Eric Burns, study co-author
and astrophysicist at Louisiana State University in Baton Rouge.
How could gold be made at a magnetar?
Neutron stars are the collapsed cores of stars that have exploded. They are so
dense that one teaspoon of neutron star material, on Earth, would weigh as
much as a billion tons. A magnetar is a neutron star with an extremely powerful
magnetic field.
On rare occasions, magnetars release an enormous amount of high-energy
radiation when they undergo “starquakes,” which, like earthquakes, fracture the
neutron star’s crust. Starquakes may also be associated with powerful bursts of
radiation called magnetar giant flares, which can even affect Earth’s
atmosphere. Only three magnetar giant flares have been observed in the Milky
Way and the nearby Large Magellanic Cloud, and seven outside.
Patel and colleagues, including his advisor Brian Metzger, professor at Columbia
University and senior research scientist at the Flatiron Institute in New York,
have been thinking about how radiation from giant flares could correspond to
heavy elements forming there. This would happen through a “rapid process” of
neutrons forging lighter atomic nuclei into heavier ones.
Protons define the element’s identity on the periodic table: hydrogen has one
proton, helium has two, lithium has three, and so on. Atoms also have neutrons
which do not affect identity, but do add mass. Sometimes when an atom
captures an extra neutron the atom becomes unstable and a nuclear decay
process happens that converts a neutron into a proton, moving the atom forward
on the periodic table. This is how, for example, a gold atom could take on an
extra neutron and then transform into mercury.
In the unique environment of a disrupted neutron star, in which the density of
neutrons is extremely high, something even stranger happens: single atoms can
rapidly capture so many neutrons that they undergo multiple decays, leading to
the creation of a much heavier element like uranium.
When astronomers observed the collision of two neutron stars in 2017 using
NASA telescopes and the Laser Interferometer Gravitational wave Observatory
(LIGO), and numerous telescopes on the ground and in space that followed up
the initial discovery, they confirmed that this event could have created gold,
platinum, and other heavy elements. But neutron star mergers happen too late in
the universe’s history to explain the earliest gold and other heavy elements.
Recent research by co-authors of the new study — Jakub Cehula of Charles
University in Prague, Todd Thompson of The Ohio State University, and
Metzger — has found that magnetar flares can heat and eject neutron star
crustal material at high speeds, making them a potential source.
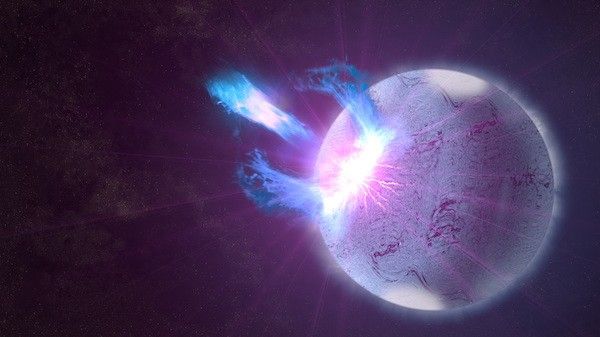
A rupture in the crust of a highly magnetized neutron star, shown here in an artist’s
rendering, can trigger high-energy eruptions.
Credit: NASA’s Goddard Space Flight Center/S. Wiessinger
New clues in old data
At first, Metzger and colleagues thought that the signature from the creation and
distribution of heavy elements at a magnetar would appear in the visible and
ultraviolet light, and published their predictions. But Burns in Louisiana
wondered if there could be a gamma-ray signal bright enough to be detected,
too. He asked Metzger and Patel to check, and they found that there could be
such a signature.
“At some point, we said, ‘OK, we should ask the observers if they had seen
any,’” Metzger said.
Burns looked up the gamma ray data from the last giant flare that has been
observed, which was in December 2004. He realized that while scientists had
explained the beginning of the outburst, they had also identified a smaller signal
from the magnetar, in data from ESA (European Space Agency)’s
INTErnational Gamma-Ray Astrophysics Laboratory (INTEGRAL), a recently
retired mission with NASA contributions. “It was noted at the time, but nobody
had any conception of what it could be,” Burns said.
Metzger remembers that Burns thought he and Patel were “pulling his leg”
because the prediction from their team’s model so closely matched the mystery
signal in the 2004 data. In other words, the gamma ray signal detected over 20
years ago corresponded to what it should look like when heavy elements are
created and then distributed in a magnetar giant flare.
Patel was so excited, “I wasn’t thinking about anything else for the next week or
two. It was the only thing on my mind,” he said.
Researchers supported their conclusion using data from two NASA heliophysics
missions: the retired RHESSI (Reuven Ramaty High Energy Solar
Spectroscopic Imager) and the ongoing NASA’s Wind satellite, which had also
observed the magnetar giant flare. Other collaborators on the new study
included Jared Goldberg at the Flatiron Institute.
Next steps in the magnetar gold rush
NASA’s forthcoming COSI (Compton Spectrometer and Imager) mission can
follow up on these results. A wide-field gamma ray telescope, COSI is expected
to launch in 2027 and will study energetic phenomena in the cosmos, such as
magnetar giant flares. COSI will be able to identify individual elements created
in these events, providing a new advancement in understanding the origin of the
elements. It is one of many telescopes that can work together to look for
“transient” changes across the universe.
Researchers will also follow up on other archival data to see if other secrets are
hiding in observations of other magnetar giant flares.
“It very cool to think about how some of the stuff in my phone or my laptop was
forged in this extreme explosion of the course of our galaxy’s history,” Patel
said.